Students in the Department of Physics and Astronomy have many opportunities to get involved in research at any level in their education at BYU. Graduate students each complete a significant research experience, and undergraduate Physics, Physics and Astronomy, and Applied Physics majors are each required to do research leading to a thesis or capstone report, respectively. Teaching majors are also encouraged to participate also. Below we list several opportunities for research with the faculty.
Research Group Meeting Times
Research groups generally meet weekly during Fall and Winter semesters. These are generally open meetings where visitors are welcome. Some professors have individual research group meetings not listed below.
Group | Day | Time | Location |
---|---|---|---|
Acoustics | Monday | 12 pm | C261 |
Astronomy | TBD | TBD | C465 |
Atomic, Molecular, Optical | Contact individual professors | ||
Biological Physics | Contact individual professors | ||
Computational General Relativity | Thursday | 2 pm | N216 |
Computational X-ray Imaging | Monday | 12 pm | N288 |
Condensed Matter | Wednesday | 4 pm | N288 |
Laser Physics (Peatross & Ware) | Thursday | 12 pm | U160 |
Materials for Space Observatories | Friday | 3 pm | N288 |
Protein Engineering (Della Corte) | Thursday | 11 am | |
Quantum | Monday | 4 pm | N216 |
Science Education | Monday | 2 pm | N209 |
Theoretical and Mathematical | Tuesday | 3 pm | N209 |
Research Opportunities
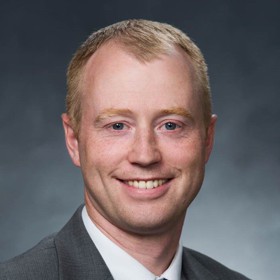
- Using time reversal of vibrations to create custom focal signals
- Time reversal acoustics to study nonlinearities in the focusing of loud sound
- Time reversal acoustic focusing of sound in the presence of a network of resonators
- Acoustic virtual reality system to assess teachers' vocal strain
- Time reversal acoustics to generate loud environments to test structures
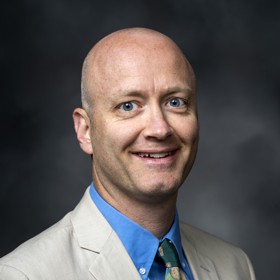
- Research in shock waves and high-amplitude acoustics
A lot of my research involves high-amplitude noise, like jets, rockets, explosions, and sonic booms. Other possibilities exist - email me to set up an appointment. Numerous publication opportunities are likely.
I'm *always* looking for new students in this area. Right now I'm looking for 2-3 students.
- Environmental Noise Monitoring and Modeling
I am working with Dr. Transtrum and graduate students on a project to use machine learning and geospatial features (nighttime radiance, precipitation, forests, etc.) to predict ambient soundscapes throughout the U.S., and eventually, globally. We have a need for 1-2 outdoor-oriented students interested in conducting making sound measurements in different urban and rural environments, formatting the data outputs, analyzing them, and helping to feed them into the machine learning models created by the graduate students.
- Machine learning and crowd noise
This project, with Dr. Transtrum, combines machine learning with crowd noise at sporting events. We want to detect cheering, booing, or even the beginning of crowd violence. This could involve taking data, analyzing them for features that relate to crowd engagement or sentiment, or developing new machine learning models.
We are currently looking for two students, one on the acoustics side for measurement and analysis, and one on the machine learning side.
- Passive acoustical monitoring of wildlife
Passive acoustic monitoring of wildlife has many purposes, but include studying population health, size, and dynamics. We're currently doing research at the Bear River Migratory Bird Refuge, looking at how water and other environmental factors impact bird choruses. We may also be starting to do rocket noise-related research - as launches can affect noise sensitive species - in California.
I'm looking for a student interested in measurements and analysis.
- Weapons noise and hearing loss
I'm working with the Office of Naval Research and Air Force Research Laboratory to develop better physics-based mathematical models for noise exposure at indoor and outdoor shooting ranges.
Why? Because 12% of marines exit basic training with significant hearing loss. This research directly protects soldiers...as they work to protect us!
Looking for a student on this project.
- Rocket noise
Dr. Grant Hart and I are studying the noise from rockets and launch vehicles. There is a LOT of interest in rockets right now as many companies around the world are developing new launch vehicle technologies. This involves potentially exciting measurements and a lot of collaborative data analysis opportunities.
We are looking for a couple students.
- Military jet noise
We study the noise radiation from turbulent jet engine exhausts. We've studied the F-22, the F-35, and the T-7A using lots of different methods for the Air Force and the Navy.
I'm looking for 1-2 students to join this group of ~5 students.
- What is PASCAL?
My research group is called PASCAL. Read about it here.

- Computational Underwater Acoustics
Large arrays of hydrophones in the ocean can be used to locate acoustic sources. The reliability of these localization algorithms depends on the degree to which the ocean environment is correctly parameterized in the models.
The computational models for sound propagation in the ocean depend on the ocean environment. My work involves using sound from the ocean to estimate the ocean environment. One part of the research works on determining the sensitivity of different seafloor parameters and determining which seafloors make a big enough difference on the sound propagation to be detected. The other part explores optimizations and how machine learning can be used to identify seafloor properties from different types of sounds.
I am currently looking for two students to join my computational underwater acoustics research. Research in this area will provide a strong foundation in computational skills, numerical modeling, and deep learning, all of which will prepare students for additional opportunities in industry, national laboratories, and graduate school.
- Underwater Acoustical Measurements
Our underwater acoustics lab (U117) has a fully automated system for making acoustic measurements in our water tank (12 ft long by 4 ft wide). Currently measurements are being made test which numerical models accurately predict sound propagation in the tank at ultrasonic frequencies. The big upcoming goal is to be able to test machine learning algorithms for source localization and environmental variability using ultrasonic tank measurements.
I am currently looking for two students to join my underwater acoustical measurement group. The opportunity to do experiments in a water tank at ultrasonic frequencies offers a solid foundation for students interested in studying ultrasound or other medical physics fields in graduate school. In addition, the experience with measurement protocols, programming the robotic arms, and analyzing the data with signal processing techniques provide a good foundation for many technical jobs.
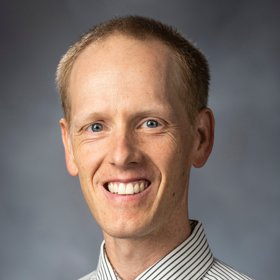
- Vibration-based Sound Power
The combination of elemental radiator theory and laser vibrometry to measure sound power of arbitrarily-shaped structures
- Physics of Percussion Instruments
The acoustic measurement and physical modeling of percussion instruments to understand and predict their sound radiation
- Signal Characterization of Seizures
the study of seizure dynamics in the brain to understand their spatial and temporal characteristics and predict the onset of severe seizure events
- Launch Vehicle Acoustics
The use of acoustic intensity in the near- and far-field of a rocket launch to localize the acoustic source
- Thermoelasticity
The study of energy dissipation mechanisms in vibrating beams and plates
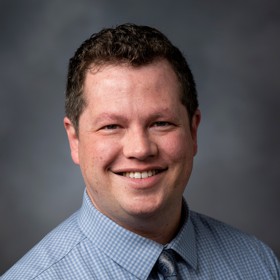
- Supermassive Black Hole Mass Measurement
The improved sensitivity and larger baselines of the Atacama Large Millimeter/submillimeter Array (ALMA) now enable imaging of molecular gas deep within the centers of numerous nearby galaxies. In a small percentage of luminous elliptical galaxies, ALMA observations trace the motion of molecules like carbon monoxide (CO) well within the supermassive black hole's (BHs) sphere of influence, wherein the BH dominates the overall gravitational potential. Using detailed gas-dynamical models, we are able to measure the BH mass and determine its overall error budget.
I am currently looking for additional students to work on this project.
- Dust Attenuation in Circumnuclear Disks
Roughly 10% of all elliptical and S0-type galaxies contain morphologically round dusty disks that obscure stellar light behind these disks. These molecular gas-rich disks are prime candidates for precision mass measurement of the supermassive black hole (BH) at the disk centers. Unfortunately, the high dust column densities preclude any confident determination of the host galaxy mass profile simply by modeling the observed stellar light distribution in optical (and often even near-IR) images. Using a large sample of multi-wavelength data obtained with the Hubble Space Telescope (HST), we are modeling the dust attenuation of these circumnuclear disks to recover a range of plausible dust-corrected stellar mass models. These will be used to more confidently constrain BH masses in about 30 elliptical and S0 galaxies.
I am currently not looking for additional students to work on this project.
- Building an Atlas of Molecular Gas Kinematics From the ALMA Archive
Archival data from the Atacama Large Millimeter/submillimeter Array (ALMA) reveal molecular gas in many early-type galaxies (ETGs, including elliptical and S0 types). In many cases, the observations trace regular rotation about the galaxy center. However, relatively few of these data sets have been published, and there is little consistency between the published data products. We are uniformly measuring gas properties in a large sample of ETGs with ALMA carbon monoxide (CO) observations, which will then be compiled into an atlas of molecular gas kinematics in luminous galaxies. In addition to measuring and analyzing kinematic properties, this atlas will also help identify candidates for higher angular resolution ALMA imaging to measure the mass of the central supermassive black hole mass.
I am currently not looking for additional students to work on this project.
- Dust Properties From ALMA Observations
Cold dust found at the centers of many nearby galaxies emits thermal radiation. With typical temperatures of 10-30 K, the dust black body spectrum peaks in the far-IR range. In many cases, the Rayleigh-Jeans tail of the dust thermal emission is detectable at mm-wavelengths with the Atacama Large Millimeter/submillimeter Array (ALMA). Together with continuum measurements from the radio to the mid-IR, data points at ALMA wavelengths are important when measuring dust mass and temperature. We are measuring ALMA continuum flux densities (or upper limits) and building spectral energy distributions (SEDs) to separate out non-thermal contributions and model the SEDs as using a modified black body function.
I am currently not looking for additional students to work on this project.
- Monitoring AGN Variability Using West Mountain Observatory
Active galactic nuclei (AGN) are electromagnetic phenomenon arising from material accreting onto supermassive black holes (BHs). Certain types of AGN called blazars display high levels of variability over timescales of minutes to years. Blazars are thought to be AGN seen down the jet that launches from directly about the BH. Using a rich data set of several blazars taken with our own West Mountain Observatory spanning about a decade, we aim to better characterize both long timescale and intra-night variability. This project will use both differential (aperture) photometry and, when possible, difference imaging to build detailed light curves. We will also explore possible systematic effects that impact our final light curve error budgets and more fully capture the inherent uncertainties in aperture photometry.
I am currently looking for students to work on this project.
- Reverberation Mapping in Active Galactic Nuclei
Active galactic nuclei (AGN) manifest a range of phenomenon in X-ray to radio wavelengths, from active accretion of material into a thermally hot disk about the central supermassive black hole (BH) to ionization of clouds at light-day distances to massive jets of material that produce significant radio emission. In this project, we seek to better understand the connection between brightening and dimming of thermal energy from the accretion disk and the strength of emission lines from ionized gas clouds in what is called the broad line region (BLR). As the accretion disk light produces the light that ionizes the surrounding gas clouds, changes in accretion-disk luminosity reverberate throughout the region, resulting in a time delay between AGN and BLR light curves. In collaboration with researchers at the University of Wyoming, we will employ optical imaging and spectroscopy as well as near-IR spectroscopy to map the response of a variety of BLR emission lines to the driving continuum.
I am currently looking for additional students to work on this project.
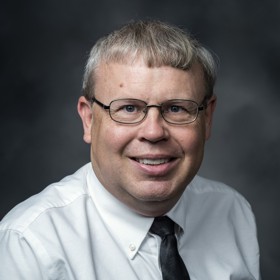
- Period Changes in Medium Amplitude delta Scuti Variables
In general, researchers consider there to be two groups of delta Scuti variables; the High Amplitude delta Scuti (HADS) and the Low Amplitude delta Scuti (LADS). However, the in between realm is interesting. The Medium Amplitide delta Scuti stars seems to show a range of changes in both amplitude and period. This makes them a very interesting group to monitor.
We are now adding some computer modeling to try to better understand these changes.
This is a place where there is always need for additional help. We will have 6 robotic telescopes running on every clear night by the summer of 2022. That means a lot of data to process and light curves to be determined.
- Variable Star Search in Open Clusters
We are currently searching for new low amplitude variable stars in a large sample of open clusters. The clusters cover a wide range of ages and will provide a evolutionary test of how the variable stars change with age. We are also looking for very small eclipses that might be the sign of a planet.
- Spectroscopic Survey of Northern Sky delta Scuti Variables
To understand the nature of the delta Scuti variables in the instability strip one needs as much information as possible about the stars. However, an examination of the catalog of delta Scuti variables shows a lack of basic information on many of the group. Of the 247 delta Scuti stars visible in the northern hemisphere we currently have spectra of 242 of them. These need to be reduced to provide estimates of some basic stellar properties like [Fe/H], radial velocity, rotational velocity, and perhaps information on any binary companions.
- Spectrophotometic Comparison of H-alpha and H-beta Index
Traditionally the H-beta index has been used as a reddening free index to measure the surface temperature of stars. Prof. Joner in the department has developed a new H-alpha index that has great promise. We are working together to spectrophotometrically compare the two systems and the application of the system to a number of different types of objects.
- Astronomy Education
I'm currently working on a project to develop a High School research competition based on pulsating variable stars. This is funded by a NASA Rocky Mountain Space Grant Consortium Mini Grant. We will provide variable star data to a number of high school teams that will then analyze the data and provide a final report that will be judged.
- Matching Model Stellar Atmosphere Models to Near-IR spectra of Pulsating Stars
The Near-IR is an area that hasn't been extensively explored for pulsating variable stars. We are obtaining NIR spectra of the stars of the instability strip (Cepheids, RR Lyrae, delta Scuti, etc.) to study a number of different issues. This includes looking for cool companion stars, molecular banding structures, line-profile changes over pulsation phases. Some of these could be related to the Hubble Tension issue.
- Impact of CO Bands in Cepheids on the Hubble Tension
We are exploring the changes of Carbon-Monoxide molecular bands over the pulsation cycle of Cepheids variables. The growth of these bands could have an impact on the Hubble Tension. This is done in the Near-IR with the ARC 3.5-m telescope. This allows complete coverage of the phase curve, which is not possible in the Mid-IR (MIR) with systems like the JWST.
- Light Curve Generation for Transiting Planets, Eclipsing Binaries, and Pulsating Variables
We have developed a number of robotic telescope systems on the observation deck of the Eyring Science Center. These range from 6" and 24". Most of the systems are currently capable of working the entire night robotically. We observe transiting planets, eclipsing binary systems, pulsating variables, and other systems that change in brightness. In some cases we really want to process the data as soon as possible to get the data sent to programs like the NASA TESS transit finding program. With the amount of data generated we just need help in processing the data. If someone were to work on this program they would get experience over a wide range of objects.
Always looking for new research students on these projects.
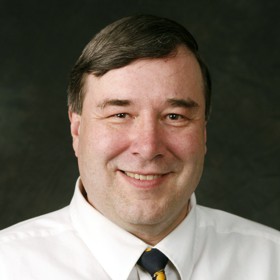
- Photometric Reverberation MappingTraditional reverberation mapping to estimate AGN black hole masses uses a combination of photometry and spectroscopy to determine the time lag between variations that occur at the accretion disk and then later in the broad line region. With such techniques, there is a need for a large amount of moderate to large telescope time in order to secure the spectroscopic data with an observing cadence suitable for a determination of the time lag. Photometric reverberation mapping uses a single epoch spectroscopic determination of the broad line region velocity and a time lag determination based on photometric observations that include predominantly continuum features or broad line components that can be seen to vary at a later time. This technique is still being tested but hold promise for the determination of black hole masses in the age of several large scale surveys.
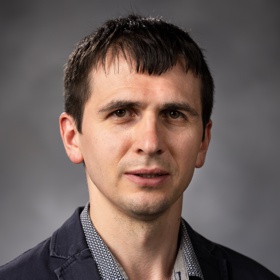
- Study of Galactic Cirrus
Join our research group to explore the mysterious Galactic cirrus—the faint, wispy clouds of interstellar dust illuminated by stars in the plane of our Galaxy or close to it, playing a crucial role in understanding the interstellar medium (ISM). By studying these clouds, you will help uncover the properties of dust grains, such as their temperature, reflectivity, and size, and distinguish them from extragalactic phenomena, enhancing the accuracy of detection and classification tasks. Leveraging ongoing and upcoming deep sky surveys like DESI Legacy, HSC-SSP, LSST, and the Roman Space Telescope, our project aims to map these clouds in unprecedented detail. With initial results already providing valuable insights, we plan to further investigate Galactic cirrus using ultradeep sky surveys and cutting-edge AI technologies, including a neural network specifically designed to identify these clouds. This is a fantastic opportunity to contribute to groundbreaking research that not only advances our understanding of the ISM but also helps in extragalactic studies. If you’re passionate about the physics of the ISM and excited by the potential of AI in astrophysics, we invite you to join us!
- Study of Extremely Distant Galaxies
Are you fascinated by the mysteries of the early Universe? Join our research group to explore the properties of spiral galaxies that formed less than a billion years after the Big Bang using the James Webb Space Telescope (JWST). Our project leverages JWST's unprecedented near-infrared and mid-infrared observations to study the structure of extremely distant, young galaxies—structures that are too faint to be resolved by other telescopes. Spiral arms, for example, are crucial regions of star formation and play a significant role in galaxy evolution, yet their formation mechanisms are not fully understood. Initial findings have already challenged current models of galaxy evolution, revealing young, luminous galaxies that shouldn't exist. Building on this foundation, we aim to uncover the physical conditions that shaped these early spiral galaxies, providing new insights into the Universe's history and the development of its diverse galactic populations. If you are excited about pioneering research at the forefront of astrophysics and want to be part of a project that pushes the boundaries of our understanding, we invite you to join us on this ambitious journey!
- Study of the Dust Distribution in Galaxies
Join our research group to refine our understanding of dust distribution in galaxies, including the Milky Way! Dust in the interstellar medium is crucial for many astrophysical processes, such as star formation, where it helps cool and collapse gas clouds into new stars. In this ambitious project, we will explore the 3D distribution of dust in a large sample of edge-on galaxies, viewed similarly to how we see the Milky Way. This unique perspective allows us to study the vertical distribution of dust along the galactic plane. Building on methods we've successfully applied to a handful of galaxies, we are expanding this research using the supercomputer at BYU to conduct the most comprehensive study of dust distribution to date. Additionally, we will improve our maps of dust distribution in the Milky Way at high Galactic latitudes using the latest observations. Our previous maps, recognized as some of the most precise, have set a strong foundation for this work. By joining this project, you'll be part of groundbreaking research that will enhance our understanding of galactic structures and evolution, with significant implications for both galactic and extragalactic astronomy. If you're passionate about the physics of dust and eager to make a substantial impact, we invite you to be a part of our team!
- Study of Polar-ring Galaxies
Join our research group to study the intriguing world of polar-ring galaxies, unique cosmic structures that serve as natural laboratories for exploring the mysteries of dark matter and galaxy formation. A polar-ring galaxy features a ring of gas, dust, and stars orbiting perpendicular to its main body, offering insights into matter accretion and the shape of the dark matter halo—a key component that influences galaxy rotation and formation. Our recent findings suggest that these galaxies are more common than previously thought, opening the door to a comprehensive study of their properties. By leveraging neural networks and deep sky surveys, we aim to identify thousands of polar-ring galaxies, greatly expanding the current known sample. This project is already underway with the help of my graduate and undergraduate students, and we invite new students to participate, providing a unique opportunity to engage with cutting-edge research. This work will not only deepen our understanding of galaxy evolution but also contribute to the broader effort to unravel the nature of dark matter, offering valuable constraints that can refine existing models and theories. If you are eager to explore these cosmic mysteries and gain hands-on experience in astrophysical research, we welcome you to join our group!
- Twins of the Milky Way Galaxy
Join our research group to search for the twins of our Milky Way galaxy using modern deep sky surveys! This project is crucial for gaining insights into the formation and evolution of spiral galaxies, including the processes that have shaped the Milky Way over billions of years. By identifying galaxies that closely resemble our own, we aim to better understand the dynamics, structure, and star formation history characteristic of the Milky Way. Utilizing data from ongoing and upcoming surveys like DESI Legacy, HSC-SSP, LSST, and the Roman Space Telescope, we will identify and analyze galaxies with similar properties to our own. A recent pilot study, conducted by my former undergraduate student, compared the Milky Way with ~6,000 edge-on galaxies. In our next phase, we will expand this comparison to ~100,000 edge-on galaxies using advanced analyses and a specially trained neural network. This research will place our Galaxy in a broader cosmic context, revealing patterns and differences that highlight the environmental factors and internal processes shaping galactic development. If you're excited about exploring our Galaxy and how it compares with other galaxies, we invite you to join us!
- The Use of Cosmological Simulations of the Universe
Join our research group to explore the Universe by comparing real galaxies observed in deep sky surveys with galaxies modeled through cutting-edge cosmological hydrodynamical simulations! These simulations use the laws of physics to model the formation and evolution of galaxies over billions of years, incorporating effects like gravity, gas dynamics, and star formation. This project is crucial for testing and refining our theoretical models by comparing them with actual observations, allowing us to identify similarities and discrepancies that improve our understanding of the fundamental processes shaping galaxies. Through this work, we aim to determine how well our current theories match reality, guiding future improvements in the models and leading to a more accurate picture of the Universe’s history and evolution. If you are passionate about galaxy modeling and want to contribute to the forefront of scientific discovery, we invite you to join our team and be part of a project that bridges the gap between theory and observation!
- Archeoastronomy
Join our research group as we collaborate with the Archaeological Research Institute (ARI) on an exciting project that merges astronomy with archaeology! We are working alongside Richard Hauck to co-author publications in archeoastronomy, drawing on nearly forty years of ARI's extensive fieldwork. Our research will cover ancient astronomical observatories on the Colorado Plateau and recent findings from Khor Kharfot in eastern Arabia. These manuscripts, which document and analyze archaeological sites with lunisolar and stellar orientations, represent decades of detailed research using computerized data techniques from the late 20th century. Our goal is to preserve and publish these valuable findings, ensuring that this knowledge is accessible to scholars and not lost in archives. This collaboration holds immense potential to unveil fascinating insights into ancient cultures, religions, and their advanced understanding of the stars. If you are intrigued by the intersection of astronomy, archaeology, history, and religion, we invite you to join us in this unique and enriching project!
- Developing New Astronomical Software
Join our research group to contribute to the development and enhancement of essential Python tools for the astronomical community! We are currently updating the IMAN Python package, widely used for data reduction and analysis of galaxies. This updated package will empower researchers in extragalactic astronomy to handle vast amounts of data more efficiently, driving more accurate and impactful scientific discoveries. By joining our team, you'll help refine this powerful tool to support the astronomical community by enhancing data analysis. If you’re passionate about coding, astronomy, and making a difference in the field, we invite you to be part of our team!
- Study of the Low-surface Brightness Universe
Join our research group to explore the low-surface brightness Universe using the upcoming Vera Rubin Telescope (LSST), which is set to revolutionize our understanding of the faint cosmos. As the most ambitious project in our group, this study will utilize LSST’s unparalleled sensitivity and the world’s largest camera to detect extremely faint structures that ordinary observations miss. Our research will focus on groundbreaking investigations, including studying tidal interactions of galaxies, examining galaxy groups and clusters, and exploring low-surface brightness and ultra-diffuse galaxies. These observations will deepen our understanding of galaxy interactions, the distribution of matter and dark matter, and the evolution of the Universe over time. By illuminating the "dark side" of the Universe, this project will provide new insights into the mechanisms shaping cosmic structures. If you're eager to be part of cutting-edge research that pushes the boundaries of our knowledge, we invite you to join us in this exciting journey and contribute to advancing our understanding of the Universe's hidden depths!
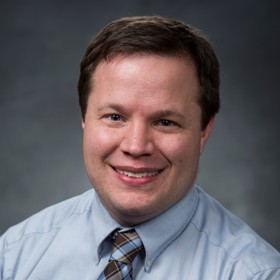
- Studying the Architectures of Exoplanetary Systems
(No positions open until Fall 2023.) Like our Sun, other stars are known to host planetary systems. As we continued to discover many more exoplanetary systems, we learn about how these systems are put together. The "architecture" of these systems (are small planets on the inside or outside? how close are the planets to each other? etc.) gives us invaluable clues to the formation of planetary systems. I used state-of-the-art statistical and computational techniques to discovery new exoplanetary systems, study existing systems, and remove the biases on their properties from our limited observational methods. There are a variety of projects available at a variety of levels and you'll be paid as Research Assistants. Please contact me for more information. The best time to contact me about available positions is about 1 month before the beginning of a semester.
- Orbits in the Outer Solar System
(No positions open until Fall 2023.) Beyond the orbit of Neptune lies a population of icy bodies whose orbits can reveal unique information about how our solar system formed. This region of the solar system is called the Kuiper Belt and these small icy bodies are called Kuiper Belt Objects (KBOs or sometimes Trans-Neptunian Objects or TNOs), though some are large enough to also qualify as "dwarf planets" like Pluto and Haumea. There are multiple projects available in my research group to study KBO satellites (e.g., Haumea's moons) and KBO orbits (e.g., the Haumea and other collisional families). There are a variety of projects available at a variety of levels and you'll be paid as Research Assistants. Please contact me for more information. The best time to contact me about available positions is about 1 month before the beginning of a semester.
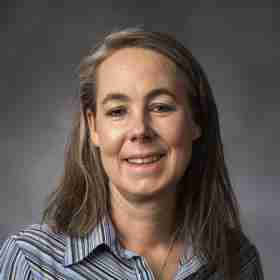
- Brown Dwarf Binary Systems - modeling
Looking at peculiarities in the spectra of known binary brown dwarfs. Trying to understand the large number of L/T transition binaries, and why the spectra of these objects change so quickly over a constant temperature range. We want to determine binary statistics with spectral type, and how many of the L/T objects are truly single objects. We want to understand which spectral features vary the most between a single brown dwarf and an unresolved binary system, so we can use these spectral features as a way to identify binaries from existing spectra. Eventually we will use high resolution photometry and psf fitting to identify marginally resolved and unresolved binaries.
- Spectrophotometry of brown dwarfs observed with ARC 3.5 meter
Use IDL to reduce spectra of brown dwarfs taken with the ARC 3.5 meter telescope.
- Variability in Brown DwarfsReduce Spitzer observations of 3 brown dwarfs taken sequentially in time to look for evidence of variability. If variability exists, the amplitude is very low. The evidence of variability would suggest that cloud features or holes in the clouds are not homogeneously distributed across the surface.
- Transiting ExoplanetsTake data with the 16" telescope on the roof of the Eyring Science Center of stars that may have transiting planets. Reduce this data using IRAF and AstroimageJ software. Characterize the radius of the planet (if we see a transit) by fitting the transit light curve. Return results to the team so that we can either obtain further observations of a possible planet candidate or expire the target as spurious or an eclipsing binary star system.
- Education - Learn Astropy and create training videos
I would love to have a student work through several tutorials I have that teach astropy, learn it very well, and then create zoom videos and jupyter notebooks that future students can use to learn how to use astropy to do photometry and spectroscopy. They could even extend this beyond to creating notebooks and videos to reduce TESS data (or any data from the roof) and in the future notebooks that can reduce APO data.
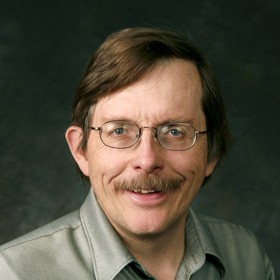
- Advanced Mirror Coatings for Hubble's successorIn 1999 the IMAGE spacecraft was successfully launched and functioned for over 6 years studying the various plasma filled regions surrounding the earth (ionosphere to magnetosphere) in wavelengths from radio through EUV. We designed and coated mirrors for the Extreme Ultraviolet Imager (EUVI) instrument, which was one of about four observational components of the IMAGE Mission. IMAGE, which stands for Imager for Magnetopause to Aurora Global Exploration, was a NASA funded Medium Explorer (MIDEX) program) [1].
We are now working with NASA scientist and engineers in the process of designing, fabricating and testing novel mirror coatings for the next generation space observatory. The flag-ship mission that comes after the James Web Telescope and WFIRST. - BYU's Entry into the University Rover ChallengeHelp prepare mars simulation rover for annual competition near Hanksville, UT June 1 http://urc.marssociety.org/home/about-urc Specifically help the science team design on-rover test for life.
- Advanced Materials for Nuclear Energy
We are able to produce as thin films existing and novel materials for nuclear energy that cannot easily made at other universities.
- Atomic layer & chemical vapor infiltration of C nanotube forests.Atomic layer and chemical vapor infiltration of carbon nanotube forests with metals such as tungsten to make three-dimensional microstructures for MEMS applications. This work is closely aligned with that of Prof. Robert C. Davis and Richard R. Vanfleet.
- Heavily doped p-type zinc oxide for UV optoelectronic devicesZinc oxide- especially heavily doped p-type material. This is a promising material for UV optoelectronics applications including UV lasers, light emitting diodes, and visible light-blind detectors. It also has applications in piezoelectricity, spintronics, transparent electronics, and as a substrate for the growth of other materials.
- optical constants of metals, semiconductors & insulators
1. we use ellipsometer in Chemistry C387 BNSN
2. we measure Optical Constants in VUV in U161 with R. Steven Turley
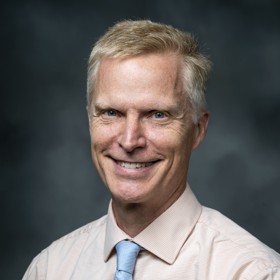
- Ultra-cold Plasmas
We are making ultra-cold plasma by photo-ionizing laser-cooled calcium atoms in a Magneto-Optical Trap (MOT). The trap size is about 1 mm and it holds about 10 million calcium atoms at a temperature of 0.001 K above absolute zero. The ultracold plasma is formed when we shine in two laser pulses that ionize all of the atoms.
The plasma is "strongly coupled", meaning that the average "nearest-neighbor" Coulomb energy is orders of magnitude larger than the mean thermal energy of particles in the plasma. A strongly coupled plasma behaves in some ways more like a solid than a gas. One of our major research goals is to understand how strong coupling changes basic processes like recombination and collisional ionization.
We use calcium to create this plasma because the energy level scheme in Ca is favorable for laser cooling and trapping. The blue wavelengths for both Ca and Ca+ are easily generated with standard laser technology. So when plasma is created we can measure the ion temperature and plasma density in a straightforward manner.
Our newest two projects are using lasers to cool the ions in the plasma, and also generating a plasma with both Ca and Yb ions at the same time. This last project will allow us to study the approach to thermal equilibrium in a two-temperature and two-mass system.
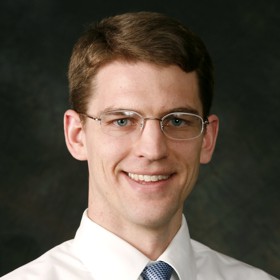
- Computing electron behavior in high-intensity laser interactions
When high-intensity lasers interact with materials, they rip electrons from atoms and pull them around at nearly the speed of light. We study electrons under these extreme conditions using MATLAB coding.
- Single photon radiation from relativistic electronsWe are building an experiment to measure the radiation produced by an accelerated electron with a large quantum-mechanical wave packet. This experiment uses extremely high intensity lasers along with single-photon detectors to study the behavior of matter at the most fundamental level.
- Measuring Nuclear Decay Rates
- Undergraduate research in string theory
Scott Glasgow (in the math department), is looking for talented undergraduate physics students interested in studying string theory. Dr. Ware will server as a co-advisor and help students fulfill the of writing a senior thesis in the department of Physics and Astronomy.

- General Group Research: Lensless imaging to study nanometer-scale material dynamics
We have positions opened for post docs, undergraduate, graduate students in the Physics and Astronomy Department. We are developing lensless or coherent diffraction imaging to study materials dynamics. We use coherent light sources (x-ray, XUV, and optical), Fourier Optics, and computer algorithms to produce nanometer scale images of materials lens-lessly.
Post doctoral, graduate and undergraduate student position projects available:
- Imaging atomic strain in structural materials
- Studying magnetic materials with tabletop extreme ultraviolet sources
- Single shot imaging of materials in extreme conditions
- 3D, lensless nanometer scale x-ray imaging via applied mathematics and data science
- Development of quantum x-ray imaging
For more information, contact Dr. Sandberg at rsandberg@byu.edu, 801-422-1497 or N261 ESC
- Imaging atomic strain in structural materials
We are studying metals at the nanometer scale to understand how and where structural materials fail. Using a lensless x-ray imaging technique known as Bragg coherent diffraction imaging, we are imaging the strain in metal grains under tension, compression, or high heat environments. This technique can currently provide 3D strain imaging at few nanometer resolution. Under support from the United States Department of Energy's Basic Energy Sciences Program, we are pushing this technique towards atomic resolution and in multiple grains.
- Single shot imaging of materials in extreme conditions
We use single, ultrafast x-ray pulses from an x-ray free electron laser to image materials while they are being shocked with high power laser systems. This research has applications to inertial confinement fusion energy applications. It is funded by the Department of Energy through SLAC National Accelerator Laboratory.
- 3D, lensless nanometer scale x-ray imaging via applied mathematics and data science
- Development of quantum x-ray imaging
New, intense and coherent x-ray sources are enabling x-ray quantum optics. In collaboration with Dr. Ryan Camacho of BYU Electrical and Computer Engineering, we are looking into the possibility of using these source to do quantum x-ray imaging including ghost imaging.
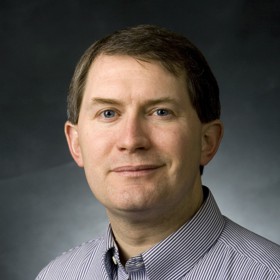
- Flexible framework materials
We use advanced mathematical tools to study the ability of crystalline inorganic materials to undergo origami-like transformations on an atomic scale, which have a profound affect on their macroscopic physical properties.
This work includes experimental, theoretical, and computational aspects. Participants have the opportunity to apply state-of-the-art research tools and methods to solve cutting-edge materials-physics problems involving superconductors, piezoelectrics, photovoltaics, spintronics, negative-thermal-expansion compounds, and catalysts.
- Topological materials
We are exploring the use of mathematical symmetry and topology to design crystal defects that endow a solid-state material with novel physical properties. This work involves theoretical and computational aspects.
This work includes theoretical and computational aspects. Participants have the opportunity to apply state-of-the-art research tools and methods to solve cutting-edge materials physics problems involving advanced functional materials such as superconductors, piezoelectrics, photovoltaics, and magnetoresistors.
- Phase transformations in crystalline materials
We develop group-theoretical tools for computing and visualizing structural distortions in crystalline materials in order to predict and understand their impact on strategic material properties.
This work includes experimental, theoretical, and computational aspects. Participants have the opportunity to apply state-of-the-art research tools and methods to solve cutting-edge materials physics problems involving advanced functional materials such as superconductors, piezoelectrics, photovoltaics, and magnetoresistors.
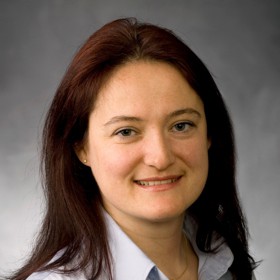
- Magnetic properties in nanomaterialsWe study magnetic properties in matter at the nanoscopic scale. Tools we use include magnetometry techniques (VSM, EHE, SMOKE), magnetic microscopy (MFM) and synchrotron techniques (XMCD, XMRS, magnetic speckles...). Types of systems we study vary from thin films (ferromagnetic, exchange bias), superparamagnetic nanoparticles, magnetically dopped materials with interesting electronic and optical properties...
- Magnetometry
We measure the magnetic response of materials to an applied magnetic field, using various types of magnetometry, including Vibrating Sample Magnetometry (VSM), Extraordinary Hall Effect (EHE) and Surface Magneto-optical Kerr Effect (SMOKE)
- Magnetic imaging
We use Magnetic Force Microscopy (MFM) to image magnetic domains in ferrmagnetic thin films. The domain pattern may vary from a stripe maze pattern to a bubble pattern depending on the magnetic history. We study in particular the effect of the magnitude of the previously applied field on the domain pattern at remanence, when the field is brought back to zero.
- X-ray magnetic scattering
We use synchrotron x-ray radiation to carry x-ray resonant magnetic scattering (XRMS) experiment at synchrotron facilities, such as NSLS, ALS APS, SLAC... The XRMS signal provides with information on the nanoscale magnetic correlations existing in the material under certain conditions of magnetic field and temperature.
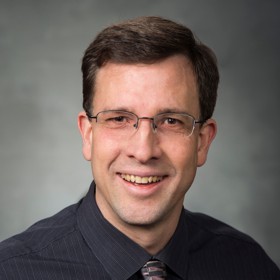
- 2D metal-halide perovskites for solar applications
"2D hybrid organic-inorganic metal halide perovskites" are a recently discovered class of semiconductors being studied in the hopes of developing highly efficient, low-cost, stable solar cells. Metal and halogen (group VII) atoms bind together in 2D layers, which are then stacked together via organic linker molecules. We are studying these interesting and important materials through optical absorption, electric field-modulated absorption, photoluminescence (fluorescence), time-dependent photoluminescence on nanosecond time scales, and dielectric spectroscopies. This allows us to determine important properties of the electrons inside these materials, to make better photovoltaic materials.
- Nanoparticles as temperature sensorsWe're working with a mechanical engineering professor (Troy Munro) to use semiconductor nanoparticles as temperature sensors. The wavelengths of light present in the nanoparticles' photoluminescence (aka fluorescence), and the time it takes for the luminescence to be emitted after the electrons have been excited both depend on the temperature. By characterizing the nanoparticles’ photoluminescence spectrum in both wavelength and time as a function of temperature, we hope to be able to use the nanoparticles as non-invasive temperature sensors in e.g. medical applications. For example, one could use the optical emission from nanoparticles injected into tissue to monitor temperatures as focused ultrasound is used to heat up and destroy tumors.

- Biomolecular ElectronicsCarbon nanotubes, proteins and nucleic acids are candidate structures for self assembled molecular electronic materials for sensing and the internet of things. .
- Nanostructures and MicromachinesWe are developing three dimensional microscale structures from vertically grown nanotube forests. We are using films of carbon atoms, few atoms thick, to make ultrastrong materials.
- Biological SeparationsThis work is focused on capture of cells and molecules using precision filters for the detection of cancer and antibiotic resistant bacteria.
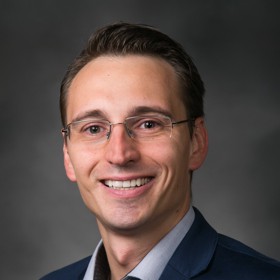
- ProSPr - Protein Structure Prediction
A cross divisional team of physicists, computer scientists, biologists and chemists implements a novel protein structure prediction pipeline to solve one of the oldest challenges in computational biophysics: The Protein Folding Problem.
We will apply our pipeline to a global community wide blind test in 2020 called CASP14.
The work entails:
- training of convolutional neural networks
- design of simulation algorithms
- high performance super computer usage
- chemical and biological evaluation of results
- Radical SAM Engineering
Together with the Chemistry department at BYU, we are developing algorithms that aid the systematic design of novel enzymes.
These enzymes can be applied to a variety of use cases, such as fertilizer production, detergent production, or drug production.
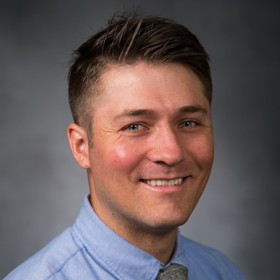
- Atomic and magnetic structure investigations of quantum materials and technologically relevant materials
One of the first steps toward understanding any given material of interest (a new superconductor, an unusual magnetic material, an energy-related compound, etc) is determining its atomic and magnetic structure. We utilize beams of x-rays, neutrons, and muons at large-scale accelerator facilities to do just that. Our primary experimental techniques include atomic and magnetic pair distribution function (PDF) analysis, conventional x-ray and neutron scattering, and muon spin relaxation/rotation. A few times a year, we visit these types of facilities to collect data, and then we come back home to analyze and make sense of it all. Through this process, we hope to shed light on the origin of the material's properties by gaining a detailed understanding of the local and average atomic and magnetic structure. If you are interested, please reach out and we can discuss if a spot is available!
- Developing open source, python-based software for investigating atomic and magnetic structure
Data are only useful if we can understand them, and to understand them, we often need specialized tools. We are currently developing open source, python-based software tools to analyze experimental data collected from condensed matter experiments using x-ray, neutron, and muon beams. The software will maximize research effectiveness and enable new methods of analysis not only for our own research group, but also for the wider community of condensed matter physicists using similar types of experimental methods. If you are interested, please reach out and we can discuss if a spot is available!
- Investigating the structure of molten salts for alternative nuclear reactor designs
Molten salt reactors (MSRs) are a promising nuclear reactor design concept in which molten ionic salts function as the coolant and/or fuel source in the reactor. MSRs have many potential advantages over standard designs in commercial use today, including greatly enhanced safety/security and the ability to produce critical medical radioisotopes in addition to vast amounts of carbon-free electricity. To make MSRs a reality, it is necessary to understand and predict the behavior of the salts in operating conditions. Gaining a detailed knowledge of the local structure of the molten salts on the atomic scale is an essential step in this direction, since the local interactions between constituent atoms determine the macroscopic properties. In this project, we use cutting-edge neutron and x-ray total scattering and computational modeling techniques to establish the structure of relevant molten salts. If you are interested, please reach out and we can discuss if a spot is available!
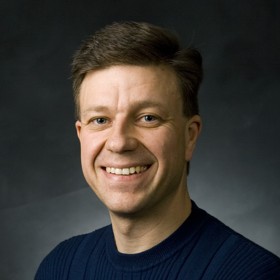
- Materials simulation: Algorithm development and applications
Our group focuses on computer simulation of materials for the purpose of discovering new materials that have exceptional properties. There are two aspects of the research: 1) developing new approaches, algorithms, and mathematics that can be used in simulation, and 2) applying existing and new approaches to find new materials. Both aspects are challenging and require participating students to move far outside their comfort zone and learn things that won't be part of a regular physics or CS degree. Students with no prior experience are welcome.
- Machine Learning for Discovering New Materials
We are developing mathematics, algorithms, and software to discover the new materials that will define the next age of human civilization. Stone, Bronze, Iron, Steel, Silcon,...what material will define the next age? We are building a "virtual laboratory" so that artificially intelligent computers can discover the materials of tomorrow. Students with no prior experience are welcome.
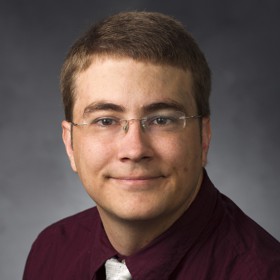
- Information theory of multi-parameter modelsMathematical modeling is a central component of nearly all scientific inquiry. Parsimonious representations of physical systems, together with robust methods for interacting with them, is one of the primary engines of scientific progress. Much of the work in our group involves developing new methods, both theoretical and computational, for improving the predictive performance of complex multi-parameter models. Our research explores the mathematical structures that enable predictive modeling. We use information theory, statistics, differential geometry, and topology, as well as relevant physical laws from a variety of fields to better understand data, models, and the relationship between reductionism and emergence.
- Computational methods for exploring high-dimensional parameter spacesModern computers enable large models of complex processes. These models often involve a large number of parameters and a relevant question is often how the model's behavior depends on the parameter values. Because the parameter space is high-dimensional, a brute force search will never be possible for models with more than a few parameters. We are developing novel computational methods for efficiently and intelligently exploring these high-dimensional parameter spaces. This project uses theoretical insights based on information theory and applies sophisticated techniques in computational differential geometry, automatic differentiation, and topology with high-performance computing. Our goal is to improve algorithms for fitting models to data, performing statistical sampling, and classifying regimes of distinct model behaviors.
- Modeling Complex Energy SystemsModels of energy systems involve a large number of heterogeneous components connected in complex networks. Detailed models of these systems constructed from physical first principles are similarly complicated and involve a large number of unknown parameters. In spite of their detail and complexity, models often have limited predictive capability because it is difficult to identify the model, i.e., find accurate values for all of the parameters. Our goals it develop models that are sufficiently complex to capture the rich behavior of real power systems, but simple enough so that all the parameters can be learned from data.
- Modeling Complex Biological SystemsBiological systems are rich in the types of behavior they can exhibit. This is enabled through a complex web of components. In the case of development biology, the relevant components are networks of chemical reactions while in neuroscience, it is a combination of electrical and biochemical signals. In both cases, the complex system responds to external stimuli and performs calculations to formulate an appropriate response. The complexity of these systems is overwhelming. New theoretical and computational tools are needed to organize our knowledge of these processes and compress it into a coherent theory. Our research tries to develop minimal models from these "parts lists" in order to summarize and organize our understanding of biological and neurological processes.
- Superconducting Materials for next generation particle acceleratorsParticle accelerators are are foundational technology in modern science, enabling fundamental research in facilities such as the Large Hadron Collider (LHC), as well as providing some of our best sources of coherent x-rays for probing nanoscale structure in materials. The same physical principles underly other technologies such as electron microscopy. Superconducting resonance cavities are the enabling technology that allows subatomic particles to be accelerated to near light speeds. In collaboration with researchers at the Center for Bright Beams (cbb.cornell.edu), we are working to better understand materials properties of superconductors in order to lay the foundation for the next generation of particle accelerators. Our work uses high performance computing to solve equations that describe how specific materials respond to applied magnetic fields, accounting for details such as surface roughness, grain boundaries, and material inhomogeneities.
- Machine Learning on Acoustic data setsSound is one of the fundamental ways we observe our environment. In collaboration with acousticians at BYU and Blue Ridge Research and Consulting, we use machine learning techniques to predict ambient sound levels from environmental parameters (such as the distance to a road or local population densities). Our models will ultimately be useful for a variety of applications including military mission planning, public health, urban development, and ecology. We also use machine learning to predict crowd dynamics from acoustic data sets. Can analysis of acoustic data collected at sporting events be used to infer the shifting mood of a diverse crowd? If so, can acoustic monitoring be used to improve law enforcement responses to crowds before they become violent?
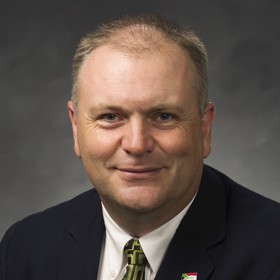
- Atomic and near atomic scale studies of materials by Transmission Electron Microscopy.We attempt to determine in as direct observational way as possible the way materials actually chose to arrange themselves. This is often in contrast to how man has attempted to arrange them. We are interested in the structural arrangement of atoms as well as the elemental and bonding arrangements of atoms within nanometer scale features of the sample. An undergraduate would learn to prepare samples for TEM analysis as well as learn the basics of TEM operation to analyze their samples in the TEM.
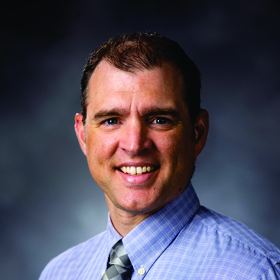
- Science Education Research
We work on science education research questions that investigate the impact our methods courses have on the beliefs and practices of future science teachers. Currently we are studying science teacher beliefs, the impacts of mentored undergraduate research opportunities, and connections between the science and engineering practices in relation to preservice teacher pedagogical content knowledge.
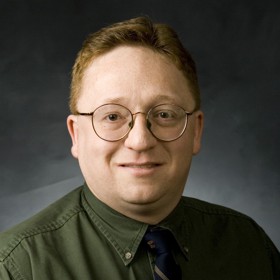
- General relativistic compact binaries
We are interested in all aspects of compact object binary mergers (black holes and neutron stars). This includes predicting the gravitational and electromagnetic radiation from such systems as well as constraining the properties of dense matter in such mergers.
This work involves large scale computation and necessitates developing numerical algorithms for solving the nonlinear partial differential equations of general relativity and radiation magnetohydrodynamics.
- Relativistic magnetohydrodynamics
Past, present and future projects include
- Establishing the characteristic structure of the equations of general relativistic magnetohydrodynamics (GRMHD) in different formulations.
- Studying the instabilities and waves associated with this system in different geometries.
- Developing constraint preserving boundary conditions for GRMHD.
- Developing simulations in 1D, 2D and 3D for flat space MHD.
- General relativisitic equilibrium models of magnetars
We would like to construct axisymmetric, general relativistic, equilibrium models of neutron stars with ultra-strong magnetic fields (magnetars). Physics inputs include poloidal and toroidal magnetic fields, realistic equations of state for the matter, differential rotation and convective motions.
- Charged black holes in higher dimensionsThe Kerr-Newman black hole is the charged, rotating black hole in 4 dimensions. The 5 dimensional version is not known. Using numerical techniques we are trying to construct it.
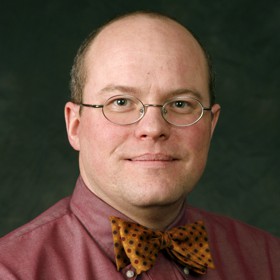
- General RelativityGeneral relativity describes gravitational phenomena geometrically as curvature in spacetime: Matter curves space, and the spacetime curvature affects matter. General relativity predicts that accelerating objects can emit gravitational radiation. While this radiation is typically extremely weak, some astrophysical systems, such as colliding black holes or neutron stars, may emit gravitational waves that we can detect on Earth. Large, kilometer scale laser interferometers, such as LIGO, are being constructed to study gravitational wave signals from these events. Unfortunately, we currently know very little about the radiation expected from the regions of spacetime with the strongest (nonlinear) gravitational fields. I study computational methods for solving the Einstein equations for these strong-field gravitational wave sources. Various projects are available to investigate black hole spacetimes, black hole formation, and properties of the Einstein equations. All projects require writing, testing, and running computer codes to investigate nonlinear gravitational phenomena.
- Relativistic fluid dynamics (RFD)Neutron star collapse, supernovae, gamma-ray sources, etc., are some of the exciting topics in relativistic astrophysics, and the perfect fluid is the fundamental model for all of these. I study relativistic perfect fluids near black holes using computational methods. In particular, Eric Hirschmann, Steven Millward and I at BYU are studying a magnetized fluid around a black hole with computational Magneto-Hydrodynamics (MHD). Various computational projects are available in RFD and MHD, which require writing, testing and running computer programs to model relativistic fluids.
- Numerical methodsResearch with the Einstein equations and RFD requires sophisticated numerical methods and techniques (as well as cheats and tricks). Some techniques include adaptive mesh refinement (AMR), parallel computing, high-resolution shock-capturing methods for fluid equations. Some systems, such as moving black holes, may naturally be solved in multiple reference frames simultaneously. I am investigating the use of overlapping computational grids for these problems. One particular interest is combining modern fluid methods with overlapping grids.
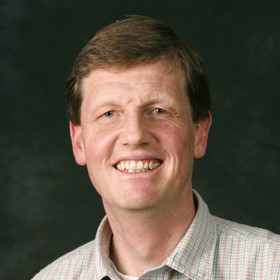
- Quantum Dynamics
Study of time evolution of quantum systems, in particular simple quantum optical systems. We are also interested in quantum clocks and their precision. The project welcomes new students. Motivation is more important than previous experience, although knowledge of linear algebra, Mathematica, and modern physics will definitely help.
- Quantum Information
Studies in entanglement, uncertainties, noise, and measurement. Applications include teleportation, cloning, and quantum algorithms, The project welcomes new students. Motivation is more important than previous experience, although knowledge of linear algebra, Mathematica, and modern physics will definitely help.
- Quantum Thermodynamics
How does thermodynamics, which traditionally studies concepts like work, heat, and entropy integrate fluctuations and new quantum resources like entanglement into its formalism? We are studying thermal machines, such as the Szilard engine in the quantum regime to probe the validity and compatibility of thermal physics, information theory and quantum theory. The project welcomes new students. Motivation is more important than previous experience, although knowledge of linear algebra, Mathematica, thermodynamics and modern physics will definitely help.
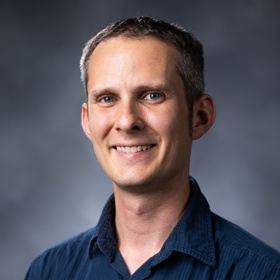
- Beyond the Standard Model phenomenology
Building models within the framework of quantum field theory to explore what nature's structure might be and how to determine that structure through experiment.
- Quantum Field Theory of Magnetic Charges
Understand how magnetic charges (monopoles) arise and behave in quantum field theory with an eye toward new experimental search strategies.
- Understanding Nontopological Solitons
Nontopological solitons are interesting objects that are possible macroscopic dark matter candidates. Making precise predictions of experimental signals depends on improving our theoretical understanding of these objects.
- Dark Matter model building
Developing models for the cosmological dark matter and determining their experimental signatures.
- Developing new models of Baryogenesis
Building models of how the Universe came to have more matter than antimatter.
- Cosmological Phase Transitions and Defects
Understand the creation and evolution of domain wall, cosmic strings, monopoles, and skyrmions in the early universe.